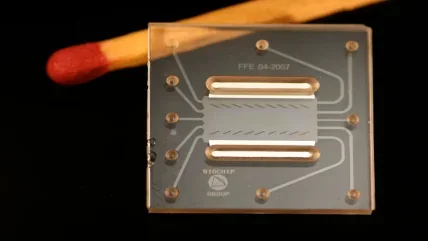
Microfluidics has long been touted as a game-changer for medical devices. While the technology has existed since the 1980s, over the past decade or so researchers have pushed the bounds of what is possible. From lab-on-a-chip diagnostics to novel techniques combining microfluidics with CRISPR-based gene editing methods, the field underpins a host of emerging applications.
As the name implies, microfluidic devices can perform fluid analysis in the realm of the very small. A chip will contain tiny microchannels, often thinner than a human hair, which enable the processing and analysis of a given liquid. You might be able to diagnose cancer using a single drop of blood, or identify Covid-19 from a single swab of the nose.
“Microfluidics came from trying to handle smaller volumes more easily and quickly than you would with most standardised equipment,” explains Nikolaj Gadegaard, professor of biomedical engineering at the University of Glasgow. “In the biomedical arena, reagents can be expensive, and you really don’t want to drain a patient of litres of blood. So small volumes have always been very desirable.”
Speed is important too. As soon as you reduce the dimensions of the systems you’re working with, you also accelerate the analysis time, simply because your molecules have a shorter distance to travel before reacting. More importantly, you can perform the tests on the spot, rather than waiting to receive results from a lab.
“Those are the two important factors that really still drive all of this,” says Gadegaard. “The chips themselves have long been touted for point of care diagnostics and point of care treatment.”
Microfluidic devices have a further advantage, in that they can test for several biomarkers simultaneously, enabling you to build up a complete picture of a disease. In this regard, microfluidics could have a key role to play within personalised medicine, for instance by stratifying cancer patients into treatment groups based on biomarker profiles.
Barriers to commercialization
This said, there remains something of a gap between the promise and the reality. For many start-ups in the field, it can be tricky to scale up production and translate a great research idea into a commercial product. As Gadegaard points out, this has to do in part with the costs of injection moulding.
“Microfluidic devices really need to be injection moulded for volume production, and the key component is not so much the machine as the tooling that is required,” he says. “Each product requires its own tooling, and I think that’s the limiting factor – every time you need to make a change to one of your devices, you need a new tool. These can cost tens to hundreds of thousands per design and device.”
Henne van Heeren, owner of enablingMNT and secretary of the Microfluidics Association, works with microfluidic start-ups to help them bring their products to market. According to his statistics, the average time needed for success at a microfluidic company is about 11 years, and $50-200m in funding is required for a new diagnostic device. It’s a protracted process with no guarantees and a lack of dedicated testing protocols.
“Two problems people have nowadays are finding the right components, and the qualification of their products by an authorised body like the FDA,” he says. “The protocols are not there. If you buy a car, you know it fulfils safety regulations, and that when you go to a petrol station, you’ll get the right petrol for your car – there’s an infrastructure behind it. All those things have yet to be developed for microfluidics.”
He remarks that, for the market to realise its potential, it needs to get easier for businesses to buy microfluidic parts off the shelf, integrate and test them. A good industry to emulate here would be consumer electronics, in which the vast majority of components used are standardised, and development is much faster.
“Saying that, I think the number of new products you can put on the market in a certain time frame in consumer electronics is much higher than in medical diagnostics,” says van Heeren. “Medical diagnostics is more conservative in that respect – it takes a long time for the doctors and hospitals to be convinced that they should implement something new here, and for the insurance companies to agree to pay the bill. Besides, the product quality needed is much higher.”
Surmounting the obstacles
An unlikely glimmer of hope came during the Covid-19 pandemic, when appetite for novel diagnostics was much greater. The microfluidics industry saw additional investments from governments and a surge of interest from venture capitalists, which went some way towards mitigating the supply chain challenges they faced.
A number of companies developed microfluidic-based tests, designed to return accurate results within minutes. For instance, Californian start-up Fluxergy raised $30m in venture funding to scale up its lab-on-a-chip testing device, while LumiraDX has developed an antigen test that verifies infection at the point of care. Researchers at the University of Bath have designed a device called LoCKamp, based on a technology called LAMP, which takes just ten minutes to perform genetic testing on a sample.
“PCR is a relatively large platform, compared to microfluidic devices, and whether it can be miniaturised entirely is a good question,” says Gadegaard. “There’s something called LAMP which is very popular. It removes a lot of the technical hardware around the cycle of genomic amplification, and can be scaled down much more easily than PCR.”
While cautioning that interest in Covid diagnostics won’t last forever, van Heeren describes the last couple of years as ‘a boon for the industry’. He thinks we might see a similar pattern with other diseases, in cases where microfluidic devices clearly outperform others on the market. That will be either in terms of the speed of returning results, or the ability to test for several parameters simultaneously.
“Microfluidics-based sepsis testing is very much of interest, because you have to define the blood biomarkers very quickly if you want to save lives,” he says by way of example.
The injection moulding obstacle may be surmountable too. Rather than using traditional techniques like CNC milling, Gadegaard’s team in Glasgow have started to 3D print the tooling used. “3D printing doesn’t require much for a new person to go in and learn – you can design the tooling, have it ready, and create your prototype devices within a day,” says Gadegaard. “That’s many, many times faster than you would get in a metal workshop. The other advantage is because we use commercial 3D printers, we can send that file to anyone else in the world, so it’s a very easy way of sharing standards and protocols. The idea was to try and lower the threshold for new people and new ideas within microfluidics.”
Since the 3D printed tooling is made from plastic, there will be some wear and tear over time compared to metal, meaning 3D printing won’t entirely replace traditional production methods. However, it gives companies much greater scope to perfect their device and make changes over time.
“I think there’s a big opportunity in the market for small volume production and rapid prototyping using this technology,” says Gadegaard. “It may well be that it can develop into something that will replace machining in the future, though the industry isn’t trained on this technology yet and the transition has yet to happen.”
Future applications
As for what else is around the corner for microfluidics, he is particularly excited about organ-on-a-chip devices. These devices, which simulate the physiology of human organs, could one day replace animal models within drug development.
“Animals are poor representatives of what humans are in terms of physiology, and it’s well known that a lot of drugs do not pass an animal test that actually could have potential for human use,” says Gadegaard. “With organ on a chip, it’s possible to humanise the technology. In other words, you would use human cells in these devices, to mimic how the drug would work in a patient. You can also start looking at rare genetic diseases, which only affect a very small population and are therefore difficult to run clinical trials for.”
On the whole, though, he thinks we need to spend less time focusing on the future possibilities, and more time acknowledging the ways that microfluidics are already being used.
“They’re more widely used than you would think,” he says. “One that came across my table the other day is just a glucose monitor you would pick up in Boots. It’s not how you might think of a microfluidic device, but it’s a small confined environment with dimensions very similar to a microfluidic device. We always keep thinking, where will this really take us, but I think it’s taken us a long way already. And it will take us much, much further in the future.”
Microfluidics, then, aren’t just the ‘next big thing’ – they’re a dominant force in the medical device industry already. And while the regulatory and manufacturing challenges should not be downplayed, neither should we underestimate the industry’s incentive to keep pushing at the frontiers of medical science.
11
The number of years, along with approximately $50-200m of funding, needed for a microfluidic company to create a new diagnostic device.
enablingMNT
Microfluidics enables multiplex PCR testing
One company in the UK has managed to integrate PCR and microfluidics for rapid point-of-care diagnostics. Co-founded by Professor Christofer Toumazou – the inventor of semiconductor-based DNA sequencing – London-based DnaNudge uses a cartridge containing 72 microwells, all of which can be spotted with different analytes to conduct multiplex testing. Initially, the company used the gadget alongside its NudgeBox – a toaster-sized PCR analyser capable of thermocycling – to detect genes linked to common diseases so that the companies app-based software could ‘nudge’ customers towards nutritional choices that reduced their risk of developing them. Since then Toumazou’s technology has played a pivotal role in containing the spread of Covid-19 on hospital wards, simply by adding the same analytes used to test for the SARS-CoV-2 virus in a lab to the DnaNudge cartridge. Microfluidics is at the heart of the process, because its what enables the mixing of swab samples and testing reagents to occur before the DNA is amplified inside a microchamber, and throughout the duration of the pandemic, the company has used the multiplexing capability of its cartridges to test multiple patients at once as well as testing for other pneumonia-causing viruses, like influenza and RSV. All the steps required to do this, which in a traditional lab would be done by hand, can take place at the micro level because of processes developed over years of study in the field of microfluidics.