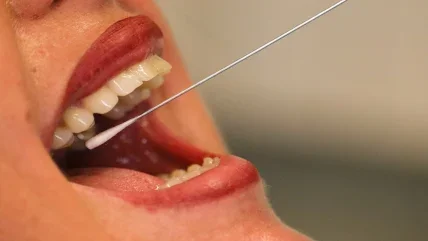
These days, most people are familiar with the PCR test given to diagnose infection with the SARS-CoV-2 virus, but many may not realise the central role that photonics technologies play in enabling it. PCR, or polymerase chain reaction, is a biomolecular method used to amplify the DNA or RNA molecule of interest. The process involves multiple amplification cycles carried out by thermal cycling. But the detection of the viral genetic material is carried out using an optical system that analyses a fluorescent tag within the mixture of sample and reagents. This tag is released only in cases where the genetic material of interest is present in the mixture, and the optical system needed to detect it requires an excitation source, a photodetector, and multiple optical filters.
The excitation sources can range from halogen lamps that emit broad-spectrum white light, to lasers that produce high-intensity light with a narrow bandwidth. While the use of laser sources is confined to collecting data from a small set of fluorophores, the halogen lamp allows a high level of multiplexing. However, to enable a halogen lamp source to analyse a broad range of fluorophores within the same system, it must be complemented with a set of narrow-bandpass filters. A common compromise is to use light-emitting diodes (LEDs).
A single LED or multiple can be present in a shuttle mechanism; arranged in an array, they can be used for individual or parallel excitation of fluorophores in the test. The detection of the signal is typically done with the use of a photodiode – a semiconductor that converts photons into an electrical current.
Perhaps the most demanding part of the optical pass in a PCR machine is the combination of multiple lenses and filters. The high degree of spectral precision is what ensures the reproducibility and reliability of the obtained results. At the excitation site, it is critical to minimise spectral overlap between fluorescent probes.
Equally critical is the optimisation of the filter sets at the detection site. Whether using several individual filters or multi-band sets, they should be optimised with respect to the excitation source. The use of multi-band sets can greatly simplify the optical path, reducing the number of filters and, therefore, the physical space requirements, but they can also compromise optimal signal detection and separation. This is one of the reasons trade-offs exist between cost, efficiency, reliability, precision, and sensitivity.
With the increased demand from test labs around the world, companies like Chroma, Iridian, Edmund Optics, Materion and Delta Optical Thin Film readily picked up on the challenge to provide custom optical filters for fluorescence-based instruments in high volumes at low cost.
Miniaturisation of testing and diagnostics devices
While PCR equipment is one of great examples of the power of photonics technologies in medical diagnostics, the pandemic made the acute need to bring testing from the central lab to the point of care more evident, making diagnostics available from the GP’s office and patients’ homes, all the way to remote locations and without easy access to healthcare centres.
Point-of-care (POC) testing devices are not a new idea, but modern technology has enabled a range of new possibilities. Developments within microfluidics, electronics, biochemistry and photonics have allowed for the development of smaller and more accurate tests. Of course, to facilitate the wide adoption of POC devices, they should be easy to use, noninvasive, accurate, user-friendly (for both patients and staff), compact, durable, and sturdy. It’s within these remits that photonics technologies are gaining a dominant role. Due to light-matter interaction, optical sensors allow for non-invasive measurement; in combination with high-sensitivity, quantitative accuracy, and the capacity for multiplexing, this makes them one of the most preferred detection mechanisms. In past years, there were challenges involved in miniaturising technology, while preserving the highest level of performance. But these are being addressed by miniaturised spectrometers, advanced coatings, micro-optics, photonics integrated circuits (PIC) and many other optical technologies that are making their way into POC diagnostics.
Photonics integrated technologies
PIC-based biosensors are optical sensors based on guided-wave integrated nanophotonics. The technology is enabling different nanometric-scale elements to guide and control light. The biosensor can be designed on a chip, facilitating high interaction between the optical field and the molecules of interest. The presence of those molecules can be tested by direct detection of the small variations in the refractive index – a measure of how much the path of light is bent, or refracted, when entering a material. The choice of material for PIC-based biosensor depends on the required passive and active elements. SiN is transparent from the visible to the mid-infrared (MIR) spectral range and allows for the measurement of various biomolecules; InP allows for the integration of active elements like high-performance amplifiers, lasers, modulators, and detectors in combination with interferometers within one chip. The integration of SiN PICs with active components based on InP can lead to high-performance systems. Furthermore, PIC technology brings possibilities for miniaturisation, extreme sensitivity, robustness, reliability, the potential for multiplexing and mass production at a low cost. Some companies, like LioniX, already have a PIC-based biosensing platform for the detection of Covid-19 and certain cancer biomarkers in its development pipeline.
Optics and spectroscopy
While miniaturisation is one of the central requirements in the development of POC testing and diagnostics devices, the performance quality of all the components must remain high. The complexity of the optical path presents a big challenge. However, micro-optics technologies have demonstrated success when integrated in many medical devices. For instance, companies like Qioptiq, an Excelitas Technology Company have extensive expertise in the design, fabrication and system integration of precision micro-optics for endoscopy, ophthalmology and surgical robotics. There are also alternative solutions for cheap optical components being developed, such as polydimethylsiloxane (PDMS) lenses. PDMS has good optical characteristics and it is heat curable, enabling good control over the curing curvature and the focal length of the lens.
PDMS lenses offer sufficient resolution for microscopic imaging. Doped with a silicone dye, PDMS lens can be used as both an emission filter and a lens, negating the need for two separate components and reducing the space required for them. Another way to reduce the space is the development of multi-purpose instruments capable of analysing multiple analytes. Since ultra-compact spectrometers detect a large spectrum – and not only a single wavelength – they are ideal as the optical transducer in multi-purpose instruments. Such spectrometers are developed, for example, by Ibsen Photonics – an OEM supplier of spectrometer modules for instrument integrators. They can deliver specialised optomechanical hardware for the multi-spectral sensor. Wasatch Photonics offers highly sensitive Raman spectrometers and systems that can be adapted for OEM use. Its integrated Raman systems for 785, 830 and 1,064nm include a Raman spectrometer with a spectral resolution of <10cm−1, laser and sampling optics. These modules are being integrated into point-of-care testing devices for non-invasive and serum-based diagnostics.
A combination of technologies
The development and production of optical biosensors and POC devices requires a combination of expertise from many different fields. For example, a typical spectroscopic unit requires the combination of fibre optics, electronics and hermetic sealing for an integrated system. The ecosystem is complex and requires significant collaboration between diverse groups from component manufactures to system integrators and end-users. To ensure the innovation behind these combined devices continues, the EU supported the creation of Europe’s first pre-commercial production line for photonics-based medical diagnostics. The EU project or Pilot Line, named MedPhab, offers a wide portfolio of photonics technologies: fibre optics, micro-optics, integrated circuits and active components. The modular technology approach allows MedPhab to simplify the development of diverse systems. The MedPhab pilot production line accelerates the commercialisation of measurement, monitoring, and diagnostic devices and treatment instruments. Seamless operation between ISO13485-certified companies and RTOs makes MedPhab a single-entry point that can serve companies working at different technology readiness levels. Furthermore, the project operates within medical domain setting requirements, which considerably accelerates the regulatory approval process.
MedPhab has already demonstrated some of its capabilities by setting up a fully automated, high-volume manufacturing process for highly sensitive plasmonic-fluidic sensors for molecular diagnostics. Companies interested in manufacturing, testing, validating or upscaling new photonics technologies for medical diagnostics can contact MedPhab directly or apply for the Demo Cases Open Call – a new programme set up to help fund chosen products. Companies developing POCT technology, looking for new partners or suppliers of photonics technologies, or just interested in learning more about the potential they have should not miss the upcoming EPIC Online Technology Meeting on Medical Devices on 6 December 2021 at 3pm CET.